|
International Nuclear Energy Academy |
HLW disposal: Status and Trends
An International Nuclear Energy Academy Statement
by
Bertrand Barré
Assisted by Dan Meneley, Dave Rossin and
Jorge Spitalnik
Introduction
High Level Waste disposal is viewed by many as
Nuclear Power’s Achilles Heel, and some people are even
convinced it constitutes an insoluble problem. It is certainly
a question about which the perception of the specialists, aware
of the progress achieved in the last two decades, differs deeply
from the perception of the public-at-large and the media. This
paper attempts to bridge part of this gap by providing up-to-date
information on the status of HLW disposal across the world.
Each country having its own classification of
radioactive waste, we shall adopt the simplest. A radioactive
substance is a substance which contains radioactive nuclei in
amount or concentration high enough to motivate radiation protection
measures. A radioactive waste is a radioactive substance resulting
from a process of human activity and which has no foreseen use
in the present technical and economic context: it must be disposed
of without harming people and environment. We shall distinguish
only three broad categories: Low level waste LLW, intermediate
level waste with long lived isotopes LL-ILW and high level waste
HLW.
LLW constitutes the bulk of the radioactive waste
in volume and in mass, but it contains only a small fraction of
the total waste radioactivity. The origin of LLW is quite diverse:
nuclear power, medicine, research, industry, etc. Many countries
have licensed operating LLW disposal sites, usually surface storage
sites which accept conditioned (immobilized) waste packages with
such specifications as to insure that within two or three centuries,
given the short radioactive period of most isotopes, the radioactivity
of the disposal site will be of the same order of magnitude as
the natural background radioactivity.

LL-ILW and HLW originate almost exclusively from
nuclear reactors and their fuel cycle facilities, as well as the
defense facilities of those countries which developed nuclear
weapons. Though quite limited in volume, they constitute the bulk
of the waste radioactivity. For those countries with no weapons
activities and which do not reprocess their spent fuel, all their
HLW and LL-ILW is inside their spent fuel assemblies which constitute
for them the ultimate waste. We shall now focus only on those
two categories of waste.
Containment, Storage, Disposal, Transmutation
For all the fear it inspires, radiation has two
precious characteristics:
-
It is easy to detect at levels far below the detection threshold
of any noxious substance (one can detect a single disintegration
when one cannot detect a given chemical unless billions of
molecules are present);
-
When detected, it is easy to protect oneself from radiation
by a combination of three ways: keeping distance, limiting
exposure time and providing shielding.
The problem of radioactive waste disposal is
therefore only a problem of containment: making sure the radioactive
species will stay where they were located, or that the migration
time from their original site to the biosphere will be long enough
for the radioactivity to have decayed much below present acceptable
limits.
The problem is exactly the same for the containment
of the radioactive elements within a nuclear reactor, but in the
case of HLW the volumic activity is far smaller, while the containment
time must be far longer. The solution, therefore, is basically
the same: containment by multiple imbedded barriers. The first
barrier is the matrix which contains the radioactive elements,
then there is the waste packaging, and then additional barriers
are added, according to the chosen disposal method.
The basic choice is between long term surface
(or subsurface) storage and deep geological disposal. Transmutation
of the longest lived elements might in the future be a preliminary
to either method.
In surface storage – sometimes called interim
storage – the conditioned waste packages are stored in engineered
facilities for a given period of time, it being clearly stated
that they will be retrieved from the facility at the end of the
specified period. The facility may be located at ground level
(surface facility) or shallowly buried (subsurface facility) in
order to improve its physical protection against external aggression.
Both surface and subsurface storage facilities must be kept under
full surveillance and monitoring during the specified period,
and one must demonstrate that the waste package can actually be
retrieved if the decision is made to do so. Interim storage provides
a satisfactory medium term solution, but it still leaves to our
successors the burden of implementing a permanent disposal solution.
In deep geologic disposal, the stratum itself
constitutes the ultimate barrier against the migration of the
radioactive elements: once full, the disposal facility will be
sealed and one does not intend to retrieve the waste packages.
Such was the initial concept, a concept put forward by the US
National Academy of Sciences as early as 1957, when asked by the
Atomic Energy Commission. More and more, in order to facilitate
public acceptance, the concept is being refined into “reversible”
geological disposal. In a reversible geological disposal, waste
packages are intended to stay, but the possibility to reverse
the decision and retrieve them is kept open for a significant
period of time, ranging from one to a few centuries. It is meant
to be a definitive solution, the best which can be implemented
today, but it does not preclude the possibility for our successors
of finding an even better solution. For practical reasons, HLW
will be held in a surface storage facility for a number of years
before being sent to geological disposal. This allows all but
the longer half-life radio-nuclides to decay, and thus the heat
source itself is substantially cooled down.
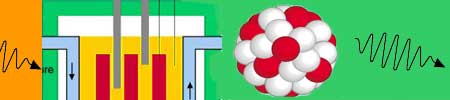
During the first few centuries, most of the radioactivity
of the waste comes from the fission products; thereafter, the
longer lived actinides (uranium, neptunium, plutonium, americium
and curium) take over. When the spent fuel is reprocessed, recovered
uranium and plutonium remain in the nuclear cycle and only traces
of them, together with the fission products and the “minor”
actinides are vitrified to constitute HLW packages. The radioactivity
of vitrified HLW decays much more rapidly than the radioactivity
of the spent fuel. If one pushes the reprocessing one step further
to recover the minor actinides (“partitioning”), curium
could be conditioned to decay by itself while neptunium and americium
could be fissioned in nuclear reactors into “ordinary”
fission products (“transmutation”). The radioactivity
of the resulting HLW packages would decay even faster, and the
necessary containment time within the disposal facility would
be reduced. This is called P&T, for partitioning and transmutation.
Implementing P&T would not eliminate the
need for ultimate disposal, but it would alleviate some design
constraints on the disposal facility. Partitioning has been developed
at the laboratory scale, and significant results have recently
been obtained. Transmutation has been demonstrated experimentally,
but present Light Water Reactors would be poor transmuters. The
high neutron fluxes inside the core of a Fast Neutron Reactor
would be much more efficient. Furthermore, a metal-fuelled Fast
Neutron Reactor with integral reprocessing and fabrication facilities
promises both high P&T efficiency and very low levels of trace
actinide materials in the waste stream. P&T is therefore a
possible useful future sophistication of the basic two methods
above described.
International Survey
As shown on the table below, which is not exhaustive, many advances
were achieved throughout the world during the last two decades:
USA |
LL-ILW |
Since 1998, a disposal site
is actually operating near Carlsbad (New Mexico): The WIPP,
a non-reversible geological disposal in a salt bed, devoted
to transuranic Defense waste disposal |
Spent Fuel |
A disposal site for spent fuel in volcanic
tuff (Yucca Mountain, Nevada) has been selected in 2002,
with Congress approval despite State opposition. Licensing
is in progress. Preliminary consideration is being given
to reprocessing the spent fuel in order to increase the
site capacity. |
Finland |
Spent Fuel |
Decision vas taken in 2001 to build a
reversible geological disposal in granite near Olkiluoto.
The site should open around 2015. An underground laboratory
ONKALO is under construction. |
Sweden |
Spent Fuel |
Site selection is almost completed for
a reversible geological disposal in granite. Target date
for operation is 2015. An underground lab has been operating
in Aspö since 1994. |
Switzerland |
HLW |
2 underground labs in granite (Grimsel)
and clay (Mont Terri) are in operation. The Swiss law stipulates
a geological disposal should open before 2040. |
Belgium |
HLW |
Many experiments have been carried out since 1984 in
the Mol underground lab (in clay). Decision for a geological
disposal site is expected in 2030.
|
Japan |
HLW |
The law voted in 2000 foresees a geological
disposal operational by 2040. JAEA has started construction
of two underground labs |
France |
HLW |
One underground lab in clay is operating.
The law voted in 2006 plans for a reversible geologic disposal
in 2015-2020 and calls for interim storage and continued
R&D on P&T. |
|
HLW |
Extensive R&D was carried out in the 70s on geological
disposal in a salt dome near Gorleben. A 10 year moratorium
was decreed in 2000 |
Spain |
Spent Fuel |
No search for a disposal site. A centralized storage
is foreseen for 2010.
|
Netherlands |
HLW |
Long-term storage in the HABOG facility. |
Canada |
Spent Fuel |
Storage was considered in 1998 “technically
acceptable, but not socially”. Disposal policy is
still under study by the government. |
UK |
LL-ILW |
Disposal policy under consideration |
Almost all countries using nuclear power have
studied geological disposal, through underground labs or “natural
analogues”, and taken part in international round robin
computer simulations. Main results show that glass and concrete,
the most extensively studied matrices for HLW and LLW containment
respectively, are durable. High integrity copper containers have
also been developed for the geological disposal of spent fuel.
If the proper site and the proper stratum are selected, the geological
barrier is very efficient at preventing radioactive nuclides migration.
While no demonstration of the behavior of a geological
disposal facility can be fully rigorous and definitive, given
the timescales involved, there are now many converging indices
that the mechanisms governing the disposal evolution in time are
understood and mastered, and that those mechanisms will induce
minimal environmental impacts.
Conclusion
We have inherited radioactive waste and we produce
it every day. We cannot simply transmit the burden to our grandchildren.
On the other hand, we must take into account a certain degree
of public mistrust of scientists and engineers when the horizon
spoken about exceeds a few centuries.
Contrary to widespread perception, a lot of progress
has been accomplished in many countries towards achieving technically
and socially acceptable HLW disposal. While there is no perfect
consensus, the majority trend is to construct geological disposal
sites, with some requirements for temporary reversibility.
Concerns about HLW management should not, therefore,
prevent mankind from pursuing the development of nuclear power.
Nuclear power and hydropower are today the only significant and
reliable sources of baseload electricity which do not originate
from fossil fuels and do not emit large amounts of gas that contribute
to the greenhouse effect.
This is an Executive Statement of the International
Nuclear Energy Academy. It represents the views of the author,
but has been endorsed by the Executive Committee of the Academy
as a contribution to the responsible development of civil nuclear
energy.
|