
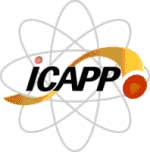
2006 International Congress on Advances in
Nuclear Power Plants
Embedded International Topical Meeting at the 2006 ANS Annual
Meeting
ICAPP '06 • June 4-8, 2006 • Reno, NV • Reno
Hilton
University of Florida
Dryout of BWR Fuel Elements
Frigyes Reisch Nuclear Power Safety, KTH Royal
Institute of Technology, Stockholm, Sweden
Phone/fax +46 8
7202365
Frigyes@safety.sci.kth.se
1. INTRODUCTION
The surface temperature of the fuel limits the power production
of nuclear reactors. Intense high temperatures can damage
the fuel cladding and cause a radioactive release and even
provoke in-vessel accident resulting in particulate debris
bed, or
core melt down. Therefore, identifying the uppermost permitted
surface temperature in a Light Water Reactor (LWR) is of
great importance. The experiments described here define
the maximum permissible power production of Boiling Water
Reactors (BWR) fuel element without the risk of burnout.
As witnessed by a great number of publications, the search
is going on for reliable criteria to assure the safety
of the fuel. Here one such criteria is analysed. Normally
the
fuel surface is effectively cooled by boiling
water.
However,
when
the heat flux exceeds a critical value the heat transfer
from the fuel surface into the coolant deteriorates, with
the result that a drastically increased fuel surface temperature
occurs. Excessive fuel temperature can be caused by overpower
or reduced coolant flow. At neutronics and thermal-hydraulic
power oscillations when the duration of the power peaks
are very short, temporary high temperature can occur without
causing fuel failures as normal cooling can quickly recover.
To avoid excessive fuel temperature, the knowledge of the
onset of the overheating phenomena is absolutely necessary,
both at the design stage and during the safe operation
of
a reactor. There are complex correlations especially developed
for specific fuel bundle designs. These correlations contains
surface power, mass flow, system pressure and other parameters.
While analyzing the test results it was recognised that
a single parameter, the void, characterises the onset
of the overheating phenomena in a wide range of pressure
and
flow conditions. These results were attained from the experimental
loop especially developed to study the dryout of BWR fuel
elements.
2. MECHANISMS OF CRITICAL HEAT FLUX
Normally the fuel surface is effectively cooled by boiling
water. However, if the heat flux exceeds a critical value
the heat transfer from the fuel surface into the coolant
that deteriorats, with the result a drastically increased
fuel surface temperature occurs. The mechanisms of critical
heat flux are:
a) Formation of hot spots under a growing bubble. Here when
a bubble grows at the heated wall a dry patch forms underneath
the bubble as the micro-layer of liquid under the bubble
evaporates. In this dry zone, the wall temperature rises
due to the deterioration in heat transfer.
b) Near-wall bubble crowding and inhibition of vapor release.
Here a “bubble boundary layer” builds up on
the surface and vapor generated by boiling on the surface
must
escape through this boundary layer. When the boundary layer
becomes too crowded with bubbles, vapor escape is impossible
and liquid cannot penetrate to the heated wall and cool
it, the surface becomes dry and overheat gives rise to
burnout.
c) Dryout under a slug or vapor clot. In plug or slug flow,
the thin film surrounding the large bubble may dry out giving
rise to localized overheating and hence burnout. Alternatively,
a stationary vapor slug may be formed on the wall with a
thin film of liquid separating it from the wall, in this
case, localized drying out of this film gives rise to overheating
and burn out.
d) Film dryout in annular flow
Figure 1. Critical Heat Flux
Mechanisms
3. EXCESSIVE FUEL TEMPERATURE
Excessive fuel temperature can be caused by overpower or
by reduced coolant flow. At thermal power and/or hydraulic
oscillations when the power peaks and/or the flow reductions
are very short and few, temporary over temperature (above
the designed limit) can occur without causing fuel failures
as normal cooling can quickly recover. To avoid excessive
fuel
temperature, which can cause damage to the fuel, knowledge
of the onset of the over heating phenomena is absolutely
necessary, both at the design stage and during the safe operation
of a reactor. There are complex correlations especially developed
for specific fuel bundle designs. These correlations contain
surface power, mass flow, system pressure and other parameters.
While analyzing the test results for a single fuel pin in
water and steam in an annular test section, it was recognized
that a single parameter, the void, is characterizing the
onset of the overheating phenomena regardless which critical
heat flux mechanism occurred.
4. MEASUREMENTS
Measurements were been carried out in a two-phase flow test
loop consisting of two heated concentric tubes, the central
one representing a fuel rod while the outer pipe emanates
the heating power corresponding to the surrounding fuel rods
in a reactor core. This loop with an anular test section
height of 7 m is presently located at the Division of Nuclear
Engineering, KTH, Royal Institute of Technology, in Stockholm
and has been in operation for some thirty years first at
the Studsvik research establishment and then at KTH to simulate
thermal hydraulic conditions in Boiling Water Reactors. (Figure
2)
Total Power: 1 MW
Total mass flow rate: 1 kg/s
Max pressure: 25 Mpa.
Max lengt of the test section: 7m.
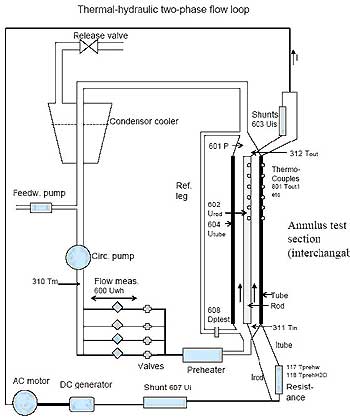
Figure 2. The loop and the test
section
5. TEST RESULTS
The results of these tests were studied to investigate
the occurrence of the onset of the excessive temperature
on the
surface of the inner and outer test tubes in this
annular flow system. The tests covered pressures of 30,
50 and 70 bar; sub-cooling 10ºC and 40ºC; mass
velocities between 250 and 2250 kg/m2s and a total
input power up to
580 kW, in this case with uniform power distribution. The
tests have been repeatedly performed in an annular test section
with a single fuel rod furnished with pin spacers, and 7
and 6 grid spacers alternatively. Then the test results were
evaluated. To calculate the steam quality, the continuity,
the heat and mass balance equations were applied. The Continuity Equations
Heat balance
Qtotal input = Qsubcooling to saturation + Qsteam building (1)
Q heat
Qsubcooling to
saturation heat used to increase
the temperature of the subcooled water to saturation temperature
Qsteam building heat used for vaporization of
part of the saturated water to steam
Mass balance
Winlet water = Wexit
water + Wexit steam (2)
W mass flow
The general definition of steam quality, sometimes called steam
value is:
x = Wsteam / ( Wwater + Wsteam) (3)
To calculate the void three known slip correlations;
Kirilov, Thoms and Zivi were used. The authors reached different
results indeed, however this does not influence the conclusions
of this paper. (Figure 3).
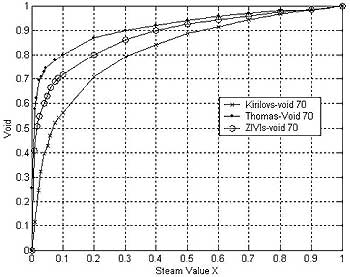
Figure3. Comparison between different void correlations as
a function of steam quality
The most important result is, that
at the onset of the excessive surface temperature the void
value changes
merely between 0.88 to 0.99, while the steam quality changes
in a wide range from 0.45 to 0.75 (Figure 4)
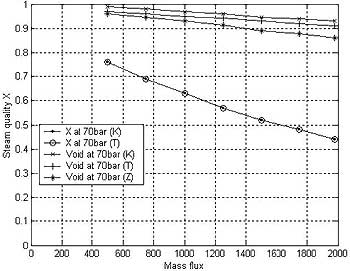
Figure 4. Void and Steam Quality as a Function
of Mass-Flow at the Onset of the Abrupt Surface Temperature
Increase
There has been knowledge of this, however
- according to this author’s but this has
not been explicitly outlined. This helps to focus
on the void when planning
further test loop experiments, as well as when
monitoring the safety of operating reactors and
when designing new fuel
assemblies. By using the constraitns described
here -limiting the permissible void content - damage
of the fuel can be avoided.
6. AVOIDING EXCESSIVE FUEL TEMPERATURE
The awareness of this result helps the design of
a tool to avoid excessive fuel surface temperature and clad
failure
in
operating
reactors. To monitor the void during operation is presently
not feasible, however from the measured parameters, power,
power distribution, coolant flow, pressure etc. the steam
quality everywhere in the core can be calculated continuously
and the void can be deduced using steam quality versus void
correlation derived from loop experiments. It is interesting
to note that an analytical model is described in the literature.
The mathematics is applied for a Freon loop and the deduced
figures coincide with the measurements from the experimental
loop mentioned above. The results are summarized in Figure
5. The abrupt increase of the temperature here too occurs
when the void value reaches around 90% for a wide range of
subcooling. 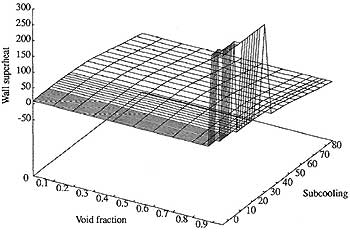
Figure 5. Prediction of critical heat flux for
Freon at p=1.5 bars, q ”= 190 kW/m2 at constant liquid
velocity of 0.5 m/
7. CONCLUSIONS
A series of experimental investigations on the maximum permissible
power production of Boiling Water Reactors (BWR) and the
effect of it on the fuel element’s surface temperature
was performed at the test facility located at KTH, Royal
Institute of Technology in Stockholm, Sweden. The
results show that the “void” is
the principal parameter for defining the onset of the excessive
surface temperature phenomena leading to burnout of a fuel
rod. |